Exploring Semiconductor Interconnection Techniques
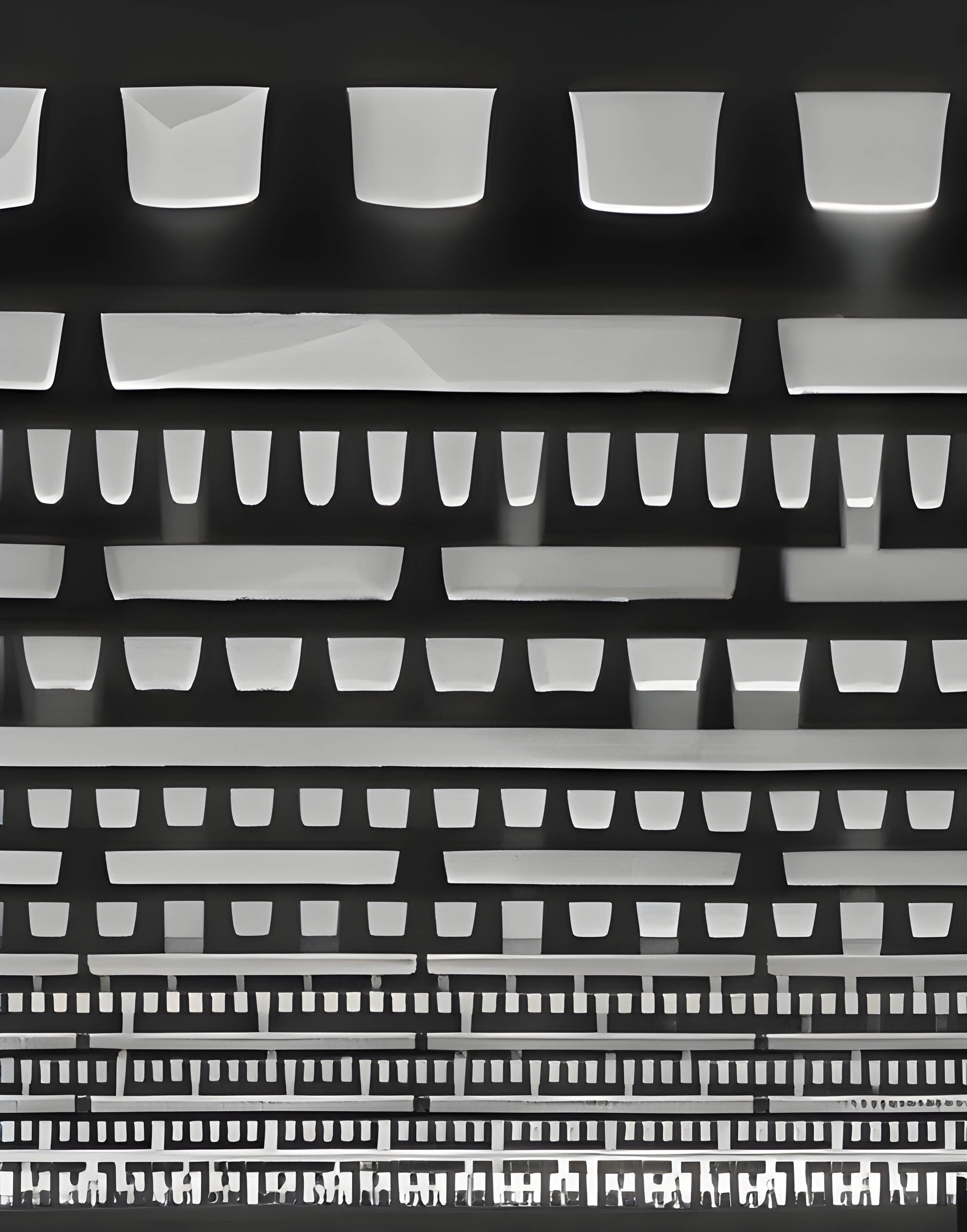
Explore the latest semiconductor interconnection techniques shaping modern technology, from metal to oxide connections, and discover future trends.
The Importance of Semiconductor Interconnection
Semiconductor interconnection plays a crucial role in the field of electronics. It allows for the seamless integration of various components and ensures efficient transmission of signals and power. Without proper interconnection techniques, the functionality and performance of semiconductor devices would be severely limited.
One of the key reasons why semiconductor interconnection is important is because it enables the creation of complex integrated circuits. These circuits consist of multiple semiconductor devices, such as transistors and diodes, interconnected to perform specific functions. The interconnections provide pathways for the flow of electrical signals between these devices, allowing them to work together harmoniously.
Another important aspect of semiconductor interconnection is its impact on device miniaturization. As technology advances, electronic devices are becoming smaller and more compact. This trend is made possible by the development of interconnection techniques that allow for the efficient routing of signals in tight spaces. By minimizing the size of interconnections, manufacturers can pack more functionality into smaller devices.
Overall, semiconductor interconnection is essential for the development of advanced electronic systems. It enables the creation of complex circuits, facilitates device miniaturization, and ensures the efficient transmission of signals and power.
Metal Interconnection Techniques
Metal interconnection techniques are widely used in the semiconductor industry due to their excellent electrical conductivity and mechanical properties. These techniques involve the deposition of metal layers, such as copper or aluminum, on a semiconductor substrate to create interconnect structures.
One common metal interconnection technique is known as damascene process. In this process, a patterned dielectric layer is first deposited on the substrate, followed by the deposition of a thin layer of metal. The excess metal is then removed using chemical mechanical polishing (CMP), leaving behind the metal interconnects.
Another popular metal interconnection technique is the dual damascene process, which allows for the simultaneous formation of interconnects and vias. Vias are vertical connections that enable the vertical integration of multiple metal layers. The dual damascene process involves the deposition of a dielectric layer with pre-defined via holes, followed by the deposition and removal of metal to form both interconnects and vias.
Metal interconnection techniques offer several advantages, including low resistance, high reliability, and compatibility with existing semiconductor manufacturing processes. However, they also face challenges such as electromigration and reliability issues at smaller feature sizes.
Despite these challenges, ongoing research and development in metal interconnection techniques continue to improve their performance and enable the advancement of semiconductor technology.
Oxide Interconnection Techniques
Oxide interconnection techniques are an alternative approach to metal interconnection in semiconductor devices. These techniques involve the use of oxide materials, such as silicon dioxide (SiO2), as interconnect structures.
One commonly used oxide interconnection technique is the shallow trench isolation (STI) process. In this process, shallow trenches are etched into the semiconductor substrate and filled with oxide material. The excess oxide is then removed, leaving behind isolated regions that can be used for interconnections.
Another oxide interconnection technique is the use of interlayer dielectric (ILD) materials. ILDs are insulating layers that separate metal interconnects from each other and from the underlying semiconductor substrate. They help to reduce cross-talk and parasitic capacitance, improving the overall performance of the device.
Oxide interconnection techniques offer advantages such as low capacitance, good isolation properties, and compatibility with existing semiconductor processes. However, they also have limitations, including higher resistance compared to metal interconnects and the need for additional manufacturing steps.
Despite these limitations, oxide interconnection techniques are being actively researched and developed to address their shortcomings and explore their potential applications in future semiconductor devices.
Future Trends in Semiconductor Interconnection
The field of semiconductor interconnection is continuously evolving, driven by the demand for higher performance, lower power consumption, and increased device integration. Several future trends can be observed in this area.
One of the key trends is the development of new materials for interconnections. Researchers are exploring alternative materials with improved electrical conductivity, lower resistance, and better reliability. These materials could potentially replace traditional metals or oxides and offer enhanced performance for future semiconductor devices.
Another trend is the miniaturization of interconnections. As devices become smaller and more compact, interconnections need to be scaled down accordingly. This trend involves the development of advanced lithography techniques, new deposition methods, and innovative manufacturing processes to create interconnect structures with nanoscale dimensions.
Furthermore, the integration of interconnections with other components is gaining importance. The ability to integrate interconnections with sensors, actuators, and other electronic components opens up new possibilities for smart and interconnected devices. This trend involves the development of heterogeneous integration techniques and the exploration of new device architectures.
In conclusion, the future of semiconductor interconnection holds great promise. Through the development of new materials, miniaturization techniques, integration strategies, and packaging advancements, interconnection technologies will continue to drive the progress of modern technology.